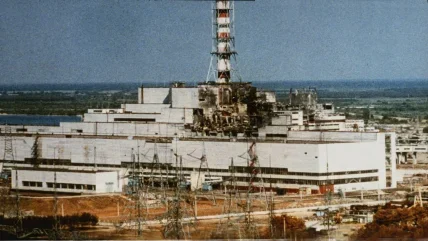
Chernobyl 4 had been due to shut down for scheduled maintenance on 26 April 1986. By noon on the day before, reactor power had been reduced to 50% and one of the two turbine generators stopped. Further reduction in reactor power was however forbidden by the grid dispatcher due to a delay in the start-up of another power plant on the grid. Resumption of the unit 4 shutdown was finally permitted by the dispatcher at 23:00 hours on 25 April.
REACTIVITY MARGIN
In accordance with normal shutdown procedures, a routine programme of tests was being carried out. The only noteworthy point on this particular occasion was that, as a result of the reactor being poisoned by xenon, the operational reactivity margin (ORM) had to be reduced to a low level. The unit computer recorded a minimum of 13.2 rods. However, at the same time the computer registered that the reactivity compensated by the automatic controller (AC) rods had not been accounted for. There were 12 such rods partly inserted into the reactor core. Therefore a minimum ORM of 15 rods was assured.
A reactivity margin of 28-32 rods was normally maintained in a stationary poisoned RBMK reactor at power, through the replacement of burnt up fuel bundles by fresh ones. The regulations contained the following restrictions: if ORM went below 26 rods, it was necessary to obtain the authorization of the chief engineer for further operation, and if ORM went below 15 rods, the reactor had to be shut down. These restrictions, which were added to the regulations after an accident at Leningrad unit 1 in 1975, were designed to enable the operator to control the power density distribution in the core. That, at least, is how the rationale for the restrictions was interpreted at the Chernobyl power station as well as at other plants equipped with RBMK reactors. There was no mention in the RBMK documentation that at low values of ORM the emergency protection system became, owing to faulty control rod design, the exact opposite of a protection system, ie a device that drove the reactor out of control.
Were the staff of the Kurchatov Institute and of the Research and Development Institute for Power Engineering (the RBMK design organization) aware of this? Apparently yes – and there are documents to prove it. But I feel they did not have a clear notion. Otherwise their inertness right up to the time of the accident looks strange, to say the least.
I am focusing here on the operational reactivity margin because it is now generally recognized that the reactor excursion on 26 April was initiated by the actuation of the reactor emergency protection system, which under the conditions of reduced operational reactivity margin did not work properly. This was due to miscalculation in the control rod design.
After passing the xenon poisoning maximum the reactivity margin began to increase and at 23:10, with the reactor at 50% power, it constituted 26 rods. The operator started to reduce power and by 24:00, when there was no change of shift, the unit parameters were: reactor power, 760MWt; ORM, 24 rods; turbine generator No 8 under load (the plant’s other turbine generator, No 7, having been shut down); all other parameters normal.
TG RUNDOWN PROGRAMME
What remained to be done was to remove electrical load from turbine generator No 8, measure idling vibrations and perform tests according to a separate programme, let us call it the “TG rundown programme.” What was this programme and what was its purpose?
Chernobyl 4 had an emergency core cooling system (ECCS) consisting of three “fast”-acting subsystems and three slower-acting subsystems. In the case of the maximum design basis accident (MDBA), involving the rupture of large diameter pipe and disconnection of the plant from the grid, the circulation of coolant is disrupted. To cool the core, the “fast”-acting subsystems of the ECCS are brought into play: two of them feed water into the core using energy derived from compressed gas in cylinders and the third uses feed pumps powered by the rotational inertia of a running down turbine generator. The feedwater pumps are intended to operate for a length of time which is sufficient to allow start up of the emergency diesels and the three slower-acting ECCS subsystems. Tests performed earlier had given unsatisfactory results and work was needed to put the finishing touches to a “rundown-unit” for the generator excitation system.
After the disaster it was widely argued that the accident occurred because of the poor quality of the preparation and implementation of the turbine generator rundown test programme. It was pointed out that safety measures were not specified. Indeed, this section of the programme did look blank. But all necessary safety measures had been performed, and were specified in the programme as preparatory work, because they had been carried out in advance.
The disconnection of the emergency core cooling system, as specified by the programme, has come in for particular criticism. In fact, this step was introduced into the programme in full accordance with the safety documents of the time, which authorized the chief engineer to disconnect the ECCS temporarily. Prof Adolf Birkhofer of the German GRS, speaking at the international congress in commemoration of Academician Andrei Sakharov in May 1991, said: “It is absolutely clear that such instructions could readily lead to errors with serious consequences and exactly that had occurred on the night before the accident.”
One cannot but agree with the first part of this assertion, and be in favour of unconditional prohibition of even temporary disconnection of the ECCS – however small the probability of occurrence of the MDBA might be in the period when the ECCS is disconnected. At the same time, however, the second part of Prof Birkhofer’s assertion is ungrounded. The disconnection of the ECCS had no influence whatsoever on the occurrence and development of the accident. Nor could the system, regardless of assertions made by Soviet delegations to the IAEA, have had any influence on the scale of the disaster. It was not designed for such an accident and it was destroyed by the explosion. There was anyway nothing for it to cool: the fuel channels had been destroyed, the fuel had been turned to ash and there was effectively no reactor left.
The carrying out of the test called for in the “TG rundown programme” also had no influence on the occurrence of the accident. The experiment required no special operating conditions at the plant. Four main circulating pumps (MCP) in each of the plant’s two loops were to be operated instead of the usual three per loop. But such operating conditions occurred frequently during pump tests, after repairs for example, and were included in the operational instructions.
Neither can the accident be attributed to lack of rigour in the operational instructions. There are no technical reasons prohibiting the operation of four main circulating pumps in each loop. The maximum coolant flow through the fuel channels is restricted only by vibration of the fuel assemblies. But on this occasion conditions were far from this. The Soviet delegation which provided information on the accident to the IAEA were however correct when they indicated in their table of operating rule violations committed by the station staff (presented at the August 1986 post-accident meeting) that the flow rate of two or three pumps out of eight was excessive. These pumps could have been torn apart because of insufficient delivery head.
And those who assert that owing to high coolant flow rate the coolant temperature at the core inlet came close to the saturation point – from which it is concluded that the loop was thermohydraulically unstable – are incorrect. This incorrect assumption roams from one document to another. It is valid only for the main coolant pump inlets, not for the core inlet.
There was also nothing remarkable about powering four of the main circulating pumps from the grid and the other four from a running down turbine generator. It was not the pumps but the head throttling valves (HTV) that were connected in parallel. The head v flow rate curve of the HTV at a low reactor power, when the head drop occurs mainly at the HTV, is not arched, but falls steeply – rendering the parallel operation mode stable. Furthermore, each pump has its own emergency protection system which shuts it off when the flow lessens. Therefore there could be no closures of non-return valves at the delivery head of pumps. However on 26 April things had not progressed that far. The monitoring system registered normal operation of all pumps at flow rates not less than 5000m3/h until the very moment when the reactor exploded. The rest of the plant was within normal limits. The emergency protection system signals will be referred to below, but they were not per se the cause of the disaster on 26 April. This is why one cannot agree with the second part of Professor Birkhofer’s assertion.
As already mentioned, the night shift accepted the reactor at a power of 760MWt. At this stage the plan called for the removal of electrical load from the generator while leaving the reactor power the same. From a technical point of view it is not very good to have high reactor power and unloaded turbine but plant staff have got to accept the situation. However, apparently following a disagreement between the unit shift foreman and the plant foreman, a reduction in reactor power was initiated. At 00:28 during the transition from local to global power control a drop of power from 520MWt down to 30-40MWt occurred. According to the operator’s entry in the log (there are no reasons not to believe it) he lowered the setpoint of the power controller, balanced the controller and turned on automatic control mode. Then he began to raise the power.
LOW POWER OPERATION
At this point it should be mentioned that there was no violation of any instructions when the staff began to reduce the power. Before the accident there were no restrictions on reactor operation at any power level. The regulations directly specify that operation at a minimum controlled power level is not limited to any particular duration. The reason why the reactor became dangerous at low power level can be readily understood from Figure 1. At low power level a given power increment results in an increase in steam volume in the coolant which is many times more than at nominal full power (Nnom). The resulting fast power coefficient of reactivity, to which the negative Doppler effect of fuel and the positive steam void effect contributed, turned out to be positive. Its specific value has been reported neither by the scientists (the Kurchatov) nor by the designers (RDIPE), although it is hard to believe that they have not calculated it after the accident. It is difficult to say whether those who created the reactor were aware of this phenomenon, which is a direct consequence of the thermal hydraulic configuration adopted. What is absolutely clear, however, is that this phenomenon was not accounted for in practice.
Figure 1. Mass (1) and volume (2) steam void coefficient of coolant v reactor power for Chernobyl 4. At low power a given power increment results in much greater voiding than when the plant is at nominal full power (Nnom)
The nuclear safety department of the power plant worked under the guidance of the above mentioned organizations and measured the fast power coefficient at power levels close to nominal full power, ie in the region where it was negative. The results were used by the operators in their everyday work. The latest data prior to the accident gave a value of minus 1.7X10-4ß/MW.
Paragraph 2.2.2. of the General Safety Provisions (OPB-82) states: “As a rule the fast power coefficient of reactivity should not be positive in any operational conditions of the nuclear power plant and under any conditions of the heat removal systems of the primary loop.” The RBMK Chief Designer, N A Dollezhal, says the following about the negative influence of large positive values of steam void coefficient of reactivity on the stability of the reactor: “…during operation with U enriched to 2% this influence is regulated by the insertion of special absorbers into the channels, as strictly specified in the operating instructions. Violations are intolerable because they make the reactor uncontrollable.” The RBMK reactor in 1986 was exactly such: enrichment 2%, no special absorbers in the core. But there were no relevant provisions in the operational instructions and nor were such operational provisions ever likely to appear since there were no references to the phenomenon in the standard reactor design documentation. And in general the reactor core was managed on the basis of calculations made by the lead design organization (RDIPE) using data obtained from the nuclear power plant itself. In other words, there were the instructions found in the operating documents on the one hand and the statement of the reactor designer on the other about procedures to make the reactor safe. But in reality the two were not properly connected and everything was done the wrong way round.
Returning to the Chernobyl case. The reactor power drop at 00:28 was nothing remarkable, it was not a rare event at all. The real essence of the matter is quite different. Did the staff violate the operational instructions when they increased reactor power after this drop? What should the staff have been guided by? Presumably by instrument readings and rules. According to the log entry, the operator raised the power using the setpoint controller and switched the power controller to the automatic mode. But under the regulations that was a partial power reduction. The only obstacle to raising power could be the drop of ORM to a value less than 15 rods. What that value really was, taking account of presently known information, one can only say after making calculations. It was impossible to measure that value at the power level which existed than at Chernobyl 4. From our knowledge of the reactor before the accident, the ORM could not have been less than 15 rods and therefore there was nothing to bar raising of the reactor power.
Recent studies of the accident, for example that of the Steinberg Commission among others, have referred to the neutron and thermal power of the reactor. These references are irrelevant because the Chernobyl 4 unit was not provided with the means for the direct measurement of thermal power. Ionization chambers and silver probe activation techniques measure only the neutron power. Thermal power is nevertheless mentioned here to distinguish it from electric power and because the system for physically monitoring the power density distribution was calibrated using thermal parameters.
After the power drop the reactor power was limited to 200MWt because that was enough for performing the required test programme. The job should have been completed in 30 minutes. The table of operating rule violations by power plant staff presented by Soviet experts to the IAEA in August 1986 includes reference to blocking the two-turbine-generator trip signal (ie the signal that shuts the reactor down when the second of the two turbines is tripped) and closing the emergency stop valve. In reality these violations did not exist. As a result of the low reactor power, pressure in the coolant loop started to fall. To stabilize the situation one could have cut off steam to the turbine, but then the two-turbine-generator emergency trip would have actuated. The shift foreman therefore turned this trip off. According to the regulations it could be turned off at a power level less than 100MWe. For the same reason the setpoint of the turbine emergency trip system was changed from 55 to 50 kg/cm2. The operator also had the right to do that. The emergency system protecting the reactor against high pressure in the loop was always turned on. Therefore the operator’s actions were logical, well grounded technically and in compliance with the requirements of the documentation then in force. The assertion contained in the table of alleged operating rule violations to the effect that the reactor emergency protection systems sensing thermal parameters had been completely turned off is a fiction. There is no need to enumerate, but the functioning of protection systems was appropriate to the operating conditions that the unit was in at the time, with the exception of protection against water level drop in the steam drums; this level was minus 1100mm instead of the minus 600mm level that would be normal for such a power level. Against the background of this information, the “international community” (the term used by one of the Soviet delegation to the IAEA post-accident meeting, Armen Abagyan) would surely have had difficulty understanding the purpose of discrediting the operating staff and how it became possible.
At 01:03 and 01:07 on 26 April main circulating pumps 7 and 8 were turned on. Measurements of turbogenerator vibration were performed and the preparatory work for carrying out tests according to the “TG rundown programme” was completed. The participating staff had been briefed and everybody went to their working stations. At that time the reactor power was 200MWt, all parameters were normal and stable, there were no emergency or warning signals, the turbine generator was powering four out of eight main circulating pumps and the feedwater pumps, while all other systems were on the reserve power supply.
The numerous judges of the operating staff have pointed out that in order to fulfil the job assigned to them the staff violated the regulations and operational instructions. But it is clear that the staff had in fact committed no violations and there were no reasons not to go through with the assigned task – bearing in mind that one must of course look at what happened against the background of the documentation which was in effect at that time and the level of knowledge about the reactor which was available to operating personnel from documents and literature.
CONTROL ROD FAULT
And yet the seeds of destruction had already been sown. For if, for any reason, a decision had been taken not to go ahead with the final test and the button had been pushed to trip the reactor or if the emergency protection system had been actuated by any signal, the explosion would have happened anyway.
With the benefit of hindsight it can be seen that there had been many cases where RBMK reactors were in a similar condition and on the brink of explosion. It turns out that the RBMK reactor, just like all nuclear reactors, was nuclear-unsafe at large values of ORM, but, unlike other reactors, it was even more dangerous at low values of ORM. What a paradox! The creators of the reactor were rather reticent about this characteristic. If they had revealed it, of course, operators would have been rather difficult to find.
Figure 2 shows schematically the design of control rods as used in the reactor control and protection system. To eliminate parasitic absorption of neutrons by the water column in a control and protection system channel when the absorbing control rod is raised, a 4.5m long graphite displacer was suspended from it. Therefore when the control rod is in contact with the upper limit switch, the displacer is positioned in the middle of the core and there are water columns 1.25m high in the upper and lower parts of the core. During the downward insertion of a rod into the upper part of the core the absorber introduces negative reactivity and the displacer, displacing water, introduces positive reactivity into the lower part. If the maximum neutron flux is shifted to the lower part of the core, then during the first 3 seconds of rod movement (rod insertion speed = 0.4 m/s) the shutdown system introduces net positive reactivity. At low values of ORM most of the control rods are out of the core and the actuation of the emergency protection system by trip signal or by pushing the trip button sends all the rods into the core.
Figure 2. The control rod arrangement at Chernobyl. The initial effect of rod insertion is to introduce positive reactivity into the bottom of the core. Key: 1 – absorber; 2 – graphite displacer; 3 – water; a – rod out of the core; b – beginning of insertion; c – sign of reactivity introduced
But owing to the core’s large height its lower and upper parts behave, especially when the central area of the core is heavily poisoned, rather like two independent reactors. The effect of the rods inserting is to create in the lower part of the core a local critical mass, and the neutron distribution tends to shift downwards irrespective of where it was before the protection system trip. The power in the lower part of the core begins to increase and then the positive fast power coefficient of reactivity comes into play. The fatal loop gets closed. Paragraph 3.3.28 of the Soviet Nuclear Safety Rules states: “The quantity, positioning and actuation speed of components of the emergency protection system must be specified and validated in the reactor design, where it should be shown that in any emergency conditions and in the event of non-actuation of the single most effective element, the actuating components of the emergency protection system provide: a rate of emergency reduction in reactor power sufficient to prevent potential damage to fuel exceeding tolerable limits: and prevention of the formation of local critical masses.” In the Chernobyl case, the safety rods were themselves creating a critical mass in the lower part of the core.
Here is what Mr Yemelyanov, deputy director of RDIPE, who directed the development of the RBMK Control and Protection System writes: “The elements for changing reactivity must be designed in such a way that a change in its direction of movement does not alter the sign of the reactivity increment.”
It is just as in the case of the steam void coefficient. On the one hand we have a document setting out the rules, while on the other hand there is the opinion of an expert as to what is required in the design of a control and protection system and … once again … things seem to have been done the wrong way round.
The Chernobyl operating staff made a mistake on 26 April in having over-looked the reduction of the ORM below the level of 15 rods stipulated in the regulations. Although the staff were not aware of the significance of the ORM in terms of its capability to transform the emergency protection system into a reactor excursion device, they were not exactly treating this parameter lightly. Controlling power density distribution is always a serious business. A violation here can lead to a major accident.
LACK OF INSTRUMENTATION
The reduction in ORM was overlooked owing to the lack of suitable instrumentation to indicate its value. The device available to the Chernobyl 4 operators at the time required about five minutes to do a single measurement and was completely useless when reactor parameters were not constant. While it was suitable for controlling the power density distribution when used in combination with the system for physically monitoring power distribution, it was completely inappropriate for the task that had just presented itself to the Chernobyl operators.
One should add that they were also getting false information about the power coefficient of reactivity. In the event of a power reduction with a negative value of the coefficient the ORM should have increased, but in reality the value of the coefficient was positive, and the operator had no way of knowing that.
To control the reactor using a system based on side ionization chambers the operator has to perform up to 1000 manipulations per hour and monitor about 4000 parameters simultaneously. In these circumstances it is somewhat cynical to accuse him of overlooking something.
The departure of ORM from normal values (by the way, 15 rods by no means guaranteed safety and it is not entirely certain that at the time of pushing the AZ-5 button the ORM was less than 15) could lead to a global-scale disaster. If that was so, then why did the designers of the reactor not fulfil the following requirements of the rules:
Paragraph 3.1.8 of the Nuclear Safety Rules, which says that, “The alarm system of the reactor must produce the following indications: signals (light and sound) when reactor parameters reach the setpoints of the emergency protection system and when reactor conditions deviate from normal; warning signals (light and sound) in the event of parameters approaching the setpoints of the emergency protection system…”
Paragraph 3.3.2 of the Nuclear Safety Rules, which says that, “The Control and Protection System must be provided with a fast acting emergency protection system securing automatic reactor trip in case of an emergency. Signals and setpoints of the emergency protection system must be validated in the design.”
Paragraph 2.7.1 of the General Safety Provisions, which stipulates that, “Protection systems must perform their functions to secure safety in case of any envisaged initiating events and in case of failures independent from the initiating event…”
A mistake by operating staff is an initiating event. Discussion about separating the functions of man and machine is clearly irrelevant here. One can talk about this when an operating error or a deviation of a parameter merely leads to unit shutdown without any damage, but no in the present case.
FINAL EVENT SEQUENCE
I feel like setting forth the final sequence of events in the first person. It had been agreed at the briefing held directly before the start of the experiment planned under the “TG rundown programme” that upon the command “start oscilloscope”: the oscillograph would be triggered to record the electrical parameters; a special additional push button designated “MPA” would be pushed to turn on the rundown-unit of the generator excitation system; steam feed to the turbine would be stopped; and the reactor would be tripped using the AZ-5 button, which is used both in emergency and normal conditions.
At 01:23:04 the monitoring system had registered the closure of the turbine stop valves. Rundown began. Everything was normal. The decrease in generator speed was accompanied by reduction in the rotational speed of the four running down main circulating pumps and the flow rate through them. The flow rate of the four main circulating pumps powered from the reserve grid supply had somewhat increased. The total flow rate of coolant had decreased by the time of the explosion down to 48-50,000m3/h. The automatic controller was holding reactor power stably while compensating positive reactivity introduced by the flow rate decrease.
It was quiet in the control room, no conversation. When someone did speak, I turned back and saw the reactor operating saying something to the shift foreman. I was about ten metres away from them and could not quite make out exactly what the operator said. The shift foreman ordered him to trip the reactor indicating with a finger: “push the button.” The foreman himself turned back to the panel he was watching. Their behaviour showed no signs of particular concern. I noted to myself that for some unknown reason the shift foreman had not given the order to trip the reactor immediately. But that did not matter at all. The reactor would have exploded 36 seconds earlier, no more than that.
I also turned to watch the instruments. The monitoring system had registered the pushing of the AZ-5 button at 01:23:40 but was not showing any warning signals at this time. These signals only started to arrive a few seconds later. At 01:23:43 the emergency signals warning of high reactor power and reduced power rise doubling period were registered. The main circulating pumps kept up a flow of coolant right up to 01:23:46, when due to a sharp power rise the flow rate through the running down main circulating pumps (loop 1) and then that through the other pumps dropped. The pressure in loop 1 went up. At 01:23:46 or 01:23:47 a large explosion was heard and, one or two seconds later, there was another one, which in my perception was even bigger. And then the silence fell.
The sequence of events seemed to be as follows:
• After pushing the AZ-5 button the emergency protection system added, according to calculations, about a ß of positive reactivity and created a local critical mass in the lower part of the core – resulting in a sharp increase of power there (see Figure 3) and the coming into play of the positive power coefficient of reactivity. The power in the lower part of the core rose to the level at which the fuel exploded and got dispersed.
Figure 3. Change in height distribution of core power density after pushing the AZ-5 button. 1,2,3 denote the distribution after three successive time intervals
• The pressure rise in the coolant, loop and the contact of coolant with fuel resulted in the rupture of the fuel channel pipework in the lower part of the reactor. The core remain without water and the steam void effect made possible a power excursion in the remaining part of the reactor, which resulted in a second explosion. I cannot tell what role the reaction between steam and zirconium played, if any.
I would like to quote here Paragraph 3.8.26 of the Nuclear Safety Rules: “The emergency protection system of the reactor must be capable of stopping the chain reaction quickly and securely in an automatic mode in the following cases: when the emergency high power setpoint is reached; when the emergency power rise rate setpoint is reached; when the reactor trip buttons are pressed.”
The way in which the Chernobyl reactor protection system fulfilled this requirement is now well known.
REAPPORTIONING BLAME
The official Soviet version of the reasons for the Chernobyl disaster, the information given by Soviet experts to the IAEA and practically all other early assessments of the accident apportioned most of the blame to the operating staff – although they could not avoid pointing to some “drawbacks” and “peculiarities” of the reactor itself.
But recently there has been an increasing tendency to recognise the “drawbacks” and “peculiarities” of the RBMK design as unacceptable. These are now seen as a root cause of the accident. However the burden of the official charges against the operating personnel seems to exert a powerful influence on people’s thinking and they are unable to abandon completely their initial perceptions. Commissions which investigated the accident or members of the International Nuclear Safety Advisory Group, who were inexplicably persuaded as to the guilt of the operators, are nevertheless not included here. True, the official Soviet spokesmen resorted to direct lies and omission of the known facts. But even so…
In conclusion:
• Whether there were operating blunders or not, it is clear to everyone that reactors capable of causing such violent explosions must never be allowed to go into operation. However good or bad the operators, there were and there will be blunders. But the possibility of errors leading to such serious consequences must be ruled out by the design features of the reactor and plant equipment.
• The Soviet regulations set out essential requirements for the design of safe reactors (as well as other equipment). They do not include any redundancy, so all the requirements must be fulfilled. The Soviet Nuclear Safety Rules and General Safety Provisions contain exact formulations in this respect. But there are several paragraphs that the Chernobyl RBMK did not comply with and some examples are quoted above. The reactor had an intolerably high positive value of steam void coefficient of reactivity which led to a positive power coefficient and to a reactivity excursion of several ß. The design suffered from dynamic instability and it was for this reason that the reactor exploded in the case of what amounted to a simulated maximum design basis accident (failure of main circulating pumps). Because of this fact alone the reactor should never have been accepted for operation.
The emergency protection system of the reactor was beneath contempt. One could perhaps sympathize with a designer if a protection system failed to cope with a large and/or rapid reactivity rise in an accident situation, but it is impossible to understand a protection system which itself drives the reactor into a power excursion. Again, this feature alone should have precluded the reactor from ever entering operation.
• At 01:23 and earlier the reactor was in a bomb-like state. An explosion might have been triggered by a trip signal actuating the emergency protection system or (as was eventually the case) by manual pushing of the scram button. And in the meantime the monitoring system issued not a single alarm signal and the operating staff did not realize the danger they were in. But not because they were blind. It might well be asked to what criteria had the monitoring systems been designed.
• The inaccurate information tolerated by the staff in performing their functions on 26 April could, had it been a reactor design which fulfilled the requirements of the regulations, led in the worst case to an unplanned trip of the reactor without any damage whatsoever.
• The time has come to say openly: the design of the RBMK-1000 was not a contributor, not a major factor, but the sole reason for the Chernobyl accident.
Author Info:
In his role as Chernobyl deputy chief engineer, Anatoly Dyatlov drew up the programme for the fateful turbine generator rundown tests on 26 April 1986. He was in the control room on the night of the accident and sustained 35% burns and a dose of around 600 rem. He was imprisoned in December 1986 and released in October 1990 on health grounds. Dyatlov always argued that he and the other operators have received an unfair share of the blame relative to the plant designers, who appear to have gone almost entirely unpunished
Related ArticlesDetails released on March SCRAM at HB Robinson 2External weblinksNuclear Engineering International is not responsible for the content of external internet sites.Focus on Chernobyl