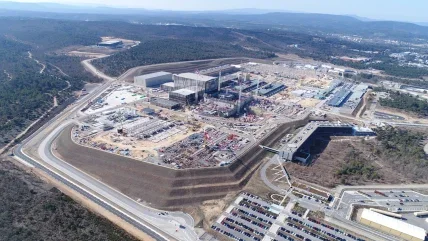
Nuclear fission and nuclear fusion have generally followed separate and sometimes competitive paths of development. While the fission community tends to support the widely-held belief that fusion “is always 30 years in the future”, the fusion community claims that fusion offers huge advantages compared with fission:
According to the ITER Organisation:
- “Fusion is a potential source of safe, non-carbon emitting and virtually limitless energy.”
- Per unit mass fusion can release nearly four times as much energy as nuclear fission reactions.
- Fusion fuels are widely available and are nearly inexhaustible. Deuterium (D) can be recovered from all forms of water, while tritium (T) may be produced during the fusion reaction as fusion neutrons interact with lithium.
- Like fission, fusion does not emit carbon dioxide or other greenhouse gases – its major by-product is helium, an inert, non-toxic gas.
- Unlike fission, nuclear fusion reactors produce no high- level activity or long-lived nuclear waste and there are no enriched materials in a fusion reactor that could be exploited to make nuclear weapons. A Fukushima-type nuclear accident is not possible in a tokamak fusion device.
- Crucially, the average cost per kilowatt hour of electricity is also expected to be similar too fission – slightly more expensive at the beginning, when the technology is new, and less expensive as economies of scale bring the costs down.
This list relates mainly to fusion produced by a tokamak device, such as that being built by ITER in southern France. Construction began in 2005 – based on a first-of-a-kind global collaboration. Europe is contributing almost half of the costs of its construction, while the other six members to this joint international venture (China, India, Japan, South Korea, Russia and the USA), are contributing equally to the rest. Tokamaks are the most common approach to confining the plasma needed for this type of fusion device. They use powerful superconducting magnets to hold the plasma in a ring-shaped (toroidal) vessel. The flow of the electrically charged plasma particles themselves also generates a magnetic field that helps to confine the plasma.
However, there are other key fusion methods. In the 1950s, US astrophysicist Lyman Spitzer showed that magnetic fields could also be configured in a twisted loop – a device known as a stellarator. Initially tokamaks, developed by the USSR in the 1960s were deemed less complex until recently. Now the Wendelstein 7-X experimental stellarator has been built in Greifswald, Germany, by the Max Planck Institute for Plasma Physics (IPP) at a cost of $1.15bn It was completed in 2015. Then there is also inertial confinement, an idea which has also been around since the 1950s where the fusion plasma is not confined by magnetic fields. Instead, a shock wave compresses it to the immense densities needed. Among others, this approach is being studied at the US National Ignition Facility (NIF) at Lawrence Livermore National Laboratory, where small capsules of D–T fuel are imploded using pulses of laser light.
A lot of work is being done at the UK Atomic Energy Agency’s (UKAEA’s) Culham Centre for Fusion Energy (CCFE), where several major projects are underway. These include the Joint European Torus (JET) which is currently the world’s biggest and most powerful tokamak. It is the focal point of the European fusion research programme which feeds into ITER. CCFE’s MAST (Mega Amp Spherical Tokamak) Upgrade is an advanced tokamak experiment trialling a design for compact fusion devices known as spherical tokamaks. Finally, the Spherical Tokamak for Energy Production (STEP) is an ambitious programme which aims to deliver an integrated design for a commercially-viable fusion power plant based on the spherical tokamak concept.
Other government supported projects include South Korea’s Korean Superconducting Tokamak Reactor (KSTAR) at the National Fusion Research Institute (NFRI) in Daejeon and China’s Experimental Advanced Superconducting Tokamak (EAST) both of which, like JET, will feed into ITER. All these projects aim to produce a sustained fusion reaction and most have achieved various levels of fusion and over periods measured in seconds. There are several ambitious plans for follow-on devices (post-2050) that will demonstrate commercial fusion energy production. These include EuroFusion’s planned successor to ITER – DEMO; South Korea’s K-DEMO (in collaboration with US Department of Energy’s Princeton Plasma Physics Laboratory) and China’s Fusion Engineering Test Reactor.
Private enterprise pile on
On top of these government initiatives, the number of private companies developing fusion concepts has grown rapidly in recent years. A survey earlier in 2022 by the Fusion Industry Association showed that investment in private fusion companies had more than doubled in the past year with eight new companies being founded, bringing the total to around 33. According to the survey, fusion companies declared more than $4.8bn of funding, up by 139% on 2021 and for the first time, private investment into fusion energy outstripped government funding.
Some private fusion companies are developing scaled down tokamak designs such as Tokamak Energy which is looking at spherical tokamaks. Commonwealth Fusion Systems (CFS) – spun out of the Plasma Science and Fusion Centre of the Massachusetts Institute of Technology (MIT) – is developing high temperature superconducting magnets for the SPARC tokamak. Another university spin out, First Light Fusion from the UK’s University of Oxford, is developing inertial confinement using an electromagnetic projectile gun instead of lasers to compress the target.
Canada’s General Fusion and UKAEA have initiated projects to advance the commercialisation of magnetised target fusion with the aim of building a demonstration machine at the Culham Campus in Oxford. This uses a centrifuge to spin a chamber filled with molten lead and lithium to open a cavity in the liquid metal, where the plasma sits. A piston system pumps more liquid metal into the chamber, compressing the plasma to initiate fusion. The process is repeated in pulses. TAE Technologies, a California start-up, plans to abandon D–T fuel and instead to fuse ordinary hydrogen with boron-11. Washington state start-up, Helion aims to generate electricity directly from fusion, without using the process to heat fluids and drive turbines. Helion plans to fuse a mixture of deuterium and helium-3. However, helium-3 would need to be produced by D–D fusion.
While these private companies build on decades of state investment in larger projects some, such as Tokamak Energy, CFS and GF, are also benefitting directly with investment from the UK government and the US DOE.
Fusion challenges
As research continues, there is a growing realisation that the claimed advantages fusion has over fission are not so clear cut.
Undoubtedly, fusion reactors are more intrinsically safe than fission. As the fusion process is difficult to start and maintain, there is no risk of a runaway reaction or core meltdown. Fusion can only occur under strict operational conditions and in the case of an accident or system failure, the plasma will naturally terminate, lose its energy very quickly and extinguish before any sustained damage is done to the reactor.
However, critics of fusion have indicated a number of potential problems that need to be addressed: the limited supply of lithium-6, beryllium and tritium; the problem of power drain; large amounts of intermediate- and low-level wastes (ILW and LLW) generated; and cooling. They also question the claim that there is no proliferation risk.
Tritium is another key concern. While the hydrogen isotope deuterium can be extracted inexpensively from seawater, tritium is not at all readily available given a half-life of just over 12 years. It exists naturally only in trace amounts and nuclear reactors are required to produce it.
Fusion scientists aim to solve this problem by breeding the tritium they need. The high-energy neutrons released in fusion reactions can split lithium into helium and tritium if the reactor wall is lined with a lithium blanket. However, a working fusion reactor is first needed to breed tritium in this way. Currently, the world’s only commercial sources are the 19 Canada Deuterium Uranium (Candu) reactors, which each produce about 0.5 kg a year as a waste product. Half of these reactors are approaching the end of their design life. Furthermore, unless new production methods can be developed the available tritium stockpile will steadily decline as fusion reactors like ITER begins burning the fuel.
To date, tritium breeding has never been tested in a fusion reactor and its efficiency is unknown. In a recent simulation, nuclear engineers at the University of California found that, in a best-case scenario, a power-producing reactor could only produce slightly more tritium than it needs to fuel itself. Tritium leakage or prolonged maintenance shutdowns could further reduce this margin.
Daniel Jassby, who was a principal research physicist working on fusion at the Princeton Plasma Physics Lab until 1999, has detailed the problems facing fusion in several recent articles, noting that less than 10% of the injected fuel will be burned before it escapes the reacting region. “The vast majority… must therefore be scavenged from the surfaces and interiors of the reactor’s myriad sub-systems and re-injected 10-to-20 times before it is completely burned,” he says, adding that, in the two magnetic confinement fusion facilities where tritium has been used (Princeton’s Tokamak Fusion Test Reactor, and JET), approximately 10% of the injected tritium was never recovered.
As to other drawbacks, Jassby says the neutron streams produced by fusion lead directly to four problems: radiation damage to structures; radioactive waste; the need for biological shielding; and the potential for the production of weapons-grade plutonium 239 – thus adding to the threat of nuclear weapons proliferation. In addition, fusion reactors share many of the problems that also face fission, “including tritium release, daunting coolant demands, and high operating costs”. Problems unique to fusion devices include unavoidable on-site power drains that drastically reduce the electric power available for sale as well as the limited availability of tritium.
Power play
Fusion reactors consume much of the power they produce, leading to “parasitic power drain, on a scale unknown to any other source of electrical power”. Essential auxiliary systems external to the reactor must be maintained continuously even when the fusion plasma is dormant and when the fusion output is interrupted for any reason, this power must be purchased from the regional grid. Power is also needed to control the fusion plasma in magnetic confinement fusion and to ignite fuel capsules in pulsed inertial confinement fusion. If the fusion power is 300 MWe, the entire electric output of 120 MWe barely supplies on-site needs, he adds. “To have any chance of economic operation… the fusion power must be raised to thousands of megawatts so that the total parasitic power drain is relatively small”.
A fusion reactor also places place immense demands on water resources for the secondary cooling loop that generates steam, as well as for removing heat from other reactor subsystems such as cryogenic refrigerators and pumps. Parasitic electric power drain places additional demands on water resources for cooling not faced by any other types of thermoelectric power plant.
To produce usable heat, the neutron streams carrying 80% of the energy from deuterium-tritium fusion must be decelerated and cooled by the reactor structure, its surrounding lithium-containing blanket, and the coolant. The neutron radiation damage in the solid vessel wall is expected to be worse than in fission reactors. A number of components, such as the blanket and divertor, are expected to need to be replaced every 5–10 years.
In addition, while the radioactivity level per kilogram of waste would be much smaller than for fission-reactor wastes, the volume would be many times larger. There is growing international recognition that this is a problem that needs to be addressed. A study published in Nuclear Fusion in May 2022 concluded: “A waste strategy needs to be developed to mitigate the impact that the large waste volumes could have on the public perception of fusion as a viable and clean alternative source of energy.” Also, this year the UK’s Committee on Radioactive Waste Management (CoRWM) produced a preliminary position paper on the implications for decommissioning, radioactive waste management, and radioactive waste disposal associated with fusion energy. It noted: “In addition to tritium emissions and contaminated materials, there will be a need to manage radioactive materials and wastes produced by neutron activation, within regulatory controls, over the whole life cycle of a fusion reactor.”
Finally, there is the assertion that fusion does not pose a risk of nuclear weapons proliferation. Jassby points out that “the open or clandestine production of plutonium 239 is possible in a fusion reactor simply by placing natural or depleted uranium oxide at any location where neutrons of any energy are flying about”. Moreover, “a reactor fuelled with deuterium-tritium or deuterium-only will have an inventory of many kilograms of tritium, providing opportunities for diversion for use in nuclear weapons”.
Fusion learning from fission
A growing realisation of these problems, especially since the construction of ITER began in 2005, has led to an understanding that, rather than viewing fission as a competitor or “poor relation”, the fusion community could learn a lot from the decades of experience accumulated during the development of the nuclear power industry. There are synergies that could benefit both communities and common problems which could be tackled together, especially with the design and development of Generation IV advanced reactors.
Articles to this effect began to appear in journals such as Fusion Science and Technology (2005 – Synergies Between Generation-IV and Advanced Fusion Power Plant Research Programs); Fusion Engineering and Design (2006 – Synergies between FNT developments and advanced nuclear fission technologies); and Revue Générale Nucléaire (2007 – Synergies between Fission and Fusion Nuclear Energy). All of these noted the need to develop new materials, problems of safety and regulation.
The IAEA has been at the forefront of encouraging understanding of the synergies in technology development between nuclear fission and fusion for energy production, and on the long-term sustainability – including the handling of radioactive waste – and legal and institutional issues for fusion facilities.
Early in 2022 (28 February – 3 March) a Kick-off Meeting was held on the IAEA’s International Project on Innovative Nuclear Reactors and Fuel Cycles (INPRO). A follow-up meeting took place in September.
The overall objective of this inter-disciplinary study is to support the fusion community in its effort to accelerate the development and implementation of fusion-based facilities and integrated fusion-fission systems within the next decades, with the early identification of possible gaps in long-term sustainability and needed capabilities utilising INPRO assessments and analyses. The scope of work includes: review and critical analysis of previous experience in the development of national legislation and infrastructure; engagement with pioneering new fusion concepts; identification of the key issues for further analysis from the perspective of the use of INPRO tools and national approaches; and identification of relevant policy choices on a global and regional level in different scenarios.
An IAEA Nuclear Energy Series Technical report or an IAEA TECDOC Series publication will be prepared in 2024 based on the results of the study. Achievement of the overall objective will be through cooperative work on cross cutting issues performed by the IAEA and INPRO member states along with inter-departmental IAEA cooperation.
“What the INPRO study is doing is bringing together a lot of non-technical areas, learning from the experience of several of the nations that were represented,” Dr Sally Forbes from the UKAEA Culham Services Centre told NEI. “Because of the timeframe, with nuclear a lot of this work was done after the initial phases, whereas with fusion we are at a stage where we can learn now. Hopefully we can build a lot of these initial discussion into guidance to produce a common framework that can bring some harmonisation internationally at the beginning of the fusion journey rather than learning the lessons afterwards, as we did with nuclear.”
For the nuclear industry, safety, security and safeguards are very well-established processes, and IAEA guidance in these areas is accepted worldwide. However, Dr Forbes pointed out that fusion has a much lower radiological hazard profile “We probably don’t need the full-blown nuclear regulation legislation guidance for fusion,” she said, adding that regulation needs to be proportionate and enabling for a technology that generally has a lower hazard profile but is very innovative. “Obviously it takes lots of components from nuclear but a lot of it is innovative. We are looking at whether nuclear regulation is appropriate.
I am sure we will borrow lots of things from it, but many fusion nations are looking at this and perhaps deciding that we need a specific framework for fusion regulation,” says Forbes.
She noted, for example, that the UK government had recently decided that fusion in the UK will be regulated as a radiological facility and not as a nuclear facility. “It still means we have to build guidance upwards,” she added, and this was part of the discussions at the INPRO meeting.
“This will feed into the INPRO study – maybe looking at that proportionality and perhaps mixing between a nuclear framework and a radiological framework for regulating fusion.” It is about “learning the positive lessons from nuclear on how to have a very structured approach to safety and security and environmental protection but also learning where that might perhaps lead to over-engineering or extended time frames that are unnecessary for the level of hazard for fusion”.
Fusion-fission collaboration
In June, the IAEA hosted a week-long wide-ranging “Technical Meeting on Synergies Between Nuclear Fusion Technology Developments and Advanced Nuclear Fission Technologies”.
The purpose of the event was to provide a forum for exchange of information as well as present up-to-date review of activities on synergies in technology development between nuclear fission and fusion for energy production at national and international levels. The discussions will feed into an IAEA Nuclear Energy Series publication which aims to provide insight on the areas covered as well as provide examples of good practices and lessons learned and offer suggestions to accelerate the transfer of technology, knowledge, and know-how from fission to fusion.
Areas where there were deemed to be strong synergies and communalities between fusion and nuclear fission included development of fusion materials to withstand harsh service conditions and minimisation of radioactive waste hazards to reasonably achievable levels.
Areas with moderate synergies and communalities between fusion and fission included:
- Fusion materials irradiation facilities under reactor-relevant conditions;
- Development of high temperature energy conversion systems;
- Computational tools, design and safety analyses codes;
- Regulatory sized for fusion energy facilities licensing;
- Reliable and feasible fuel cycle breeder material supply (lithium and lead);
- Remote handling and in-service inspection;
- Remote controllability, radiation hardened electronics and CAD-supported operations.
In general, the meeting concluded that fusion could benefit from many relevant synergies with nuclear fission experience as well as commonalities in materials development, activated metals management, remote handling, and liquid metal. There are also development parallels between Generation IV advanced fission plants and commercial fusion facilities such as DEMO. While plant licensing and decommissioning and radwaste management for fusion may require a slightly different regulatory approach – due to the reduced hazard/risk potential – elements such as design, construction, operation and parts of the fuel cycle and plasma control for fusion facilities have many synergies with fission experience.
Luigi di Pace, a consultant who worked on Fusion at Italy’s ENEA, told NEI that he believed there were good prospects for fusion and fission to work together. He noted that the fusion community was learning from the experience of nuclear in terms of design codes, safety codes and materials. “And I think in the future there will be synergies the other way round. Not only will fission help fusion but there will be some areas of expertise in which fusion can improve and advance technology in fission, in particular with respect to Generation IV reactors that are based on advanced technologies.” He added: “I’m not sure of the timeline – who will arrive first to a commercial technology. Probably it will be fission, but in the meantime there will be a bridge as both work towards a demonstrator plant. We really do not know when this will happen, but I think it will be in the middle of this century.”
He believes the big issues and main commonalities between fusion and fission are materials and waste management as well as design codes, safety analysis codes and in service inspection. “Both fusion reactors and advanced fission reactors need to operate at high temperature. This means that materials and commercial system should be designed to operate at those high temperatures.”
Learning to manage waste
As to waste management, fission reactors will soon have to handle much more decommissioning as NPPs reach the end of their operating lives. “The real problem is not the high- level waste or used fuel but the huge amount of mildly- activated metals, and fusion may face the same problem. Unless they find some other solution, every five years the entire internals of the tokamak have to be replaced because of neutron damage, which means thousands of tonnes of material. Developing new materials can be helpful not only for fusion but also for advanced reactors, many of which will also face a large neutron flux. So there is a common interest to develop materials which are resistant to neutrons.”
Tritium can also cause damage to materials. However, another commonality is that fission is needed to produce tritium. “For example, to start DEMO we will need a few kilograms of tritium. At the moment, this can only be produced by Candu reactors. The problem is that tritium decays, so you cannot store it for 20 years – it decays every 12 years”.
Di Pace expressed some concern about the new enthusiasm for fusion sparked by the growing number of private companies in the field. “They have a lot of money and really they promise to have energy- electricity by 2030 while the governmental programmes are saying we will not have electricity before 2050. There are some doubts. They are developing new concepts for a fusion machine mainly focusing on concepts and on experiments.” At the same time, the larger government-backed projects are trying to solve the problems related to materials, fuel inventory and legal and regulatory issues.
Di Pace noted that “when there is a strong driving force and also money, that is what drives these things. So really, I am quite confident that something will happen in the next two decades.” However, he added that it was important that the ITER experiment should be successful, “otherwise we have to reconsider everything”. He added: “I hope we will have a commercial reactor no later than 2070. If all goes well DEMO will begin construction in 2040. I hope the experience of JET and ITER will also produce something.” He said he expected to see some progress by the end of the decade. “This is my hope. I spent most of my career working on this, but I am getting older and probably will not survive to see the big event. But I am happy that our younger colleagues are also very enthusiastic. It is important to engage the new generation working in nuclear in both fusion and fission.”
Author: Judith Perera